Phlebotomine sand flies - Factsheet for experts
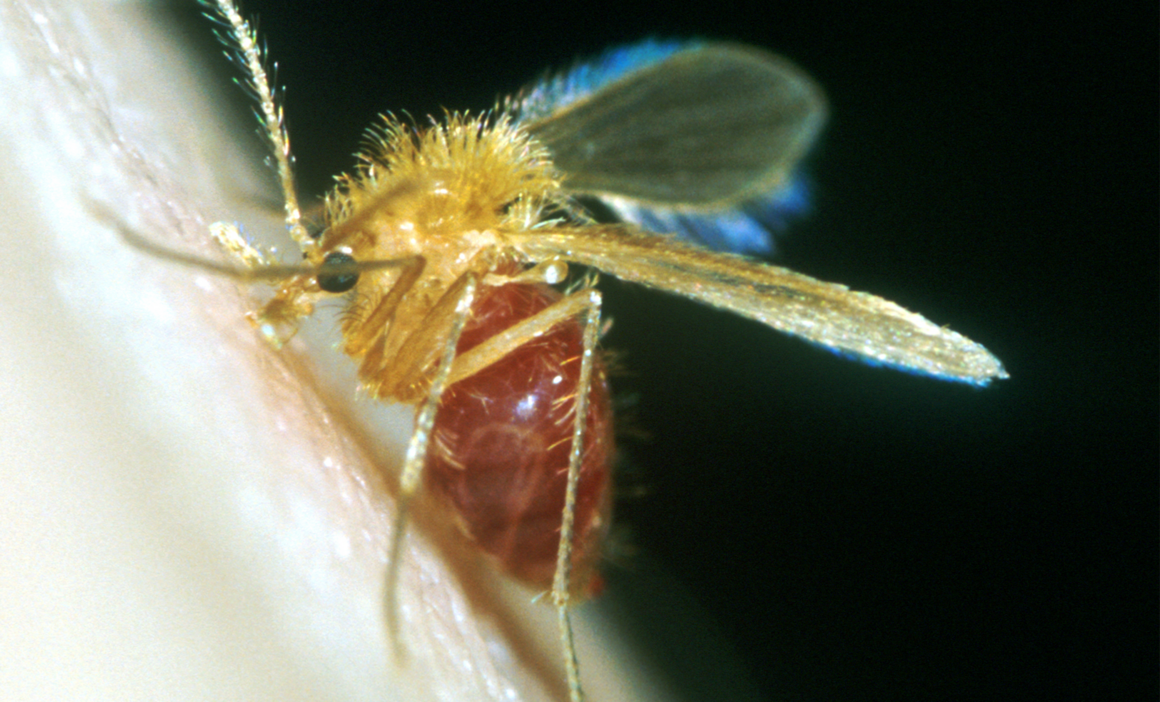
SPECIES NAME/CLASSIFICATION: Phlebotomus spp. (Diptera: Psychodidae)
COMMON NAME: Sand fly
Hazard associated with vector species
Current issues
Phlebotomine sand flies (Diptera: Psychodidae) are the natural vectors of Leishmania (Leishmania) spp. (Kinetoplastida: Trypanosomatidae) and phleboviruses (Bunyavirales: Phenuiviridae) in Mediterranean countries. Leishmania infantum and phleboviruses are widespread in the region, whereas Leishmania major and Leishmania tropica are restricted to North Africa, the Middle East and Asia Minor. Leishmania infantum causes zoonotic visceral and cutaneous leishmaniasis (VL and CL) and L. major and L. tropica zoonotic and anthroponotic CL, respectively. Phleboviruses are negative-sense RNA viruses, and most are associated with human disease causing a short, mild febrile illness known as ‘three-day fever’. One exception is Toscana virus (TOSV) which can cause a severe, and occasionally fatal, neuro-invasive disease. Although the public health impact of sand fly-borne infections is greatest in countries on the southern and eastern shores of the Mediterranean, they are an emerging problem in Europe in connection with global warming and societal behaviour that affects vector distribution and abundance. Sand flies in Europe are gradually expanding northwards and movement of dogs infected with L. infantum will probably establish new infection foci in more northerly areas where the vector is present. There is a risk of L. tropica emerging in areas of southern Europe where the vector is present, in cases among refugees and migrants from endemic peripheral countries. There are no effective vaccines to prevent sand fly-borne infections and vector control is constrained by the inherent difficulties associated with a non-aquatic, terrestrial breeding vector which is not susceptible to large-scale insecticidal treatment programmes. Europe lacks institutionalised, region-wide sand fly surveillance and control schemes for sand fly-borne pathogens. Furthermore, human leishmaniasis and TOSV infections are not included in the list of diseases under EU surveillance.
Invasive species/global dispersion
The subfamily of Phlebotomine sand flies does not include invasive species, as these fragile insects are unable to colonise new distant areas by means of passive transport. The few examples of discontinuous geographical distribution are interpreted as being due to secondary disappearance from some areas [1]. Geographical dispersion can occur to adjacent territories following environmental changes which result in suitable conditions for sand fly survival and reproduction. Of more than 50 Phlebotomus species described in Europe, North Africa, the Middle East and the Caucasus, eleven are confirmed or suspected vectors of L. infantum [2]. They include P. alexandri, P. ariasi, P. balkanicus, P. halepensis, P. kandelakii, P. langeroni, P. major, P. mascittii, P. perfiliewi, P. perniciosus and P. tobbi. Overall, P. perniciosus is the most important L. infantum vector in Europe. Phlebotomus major constitutes a complex of species; the variant described in Europe is P. neglectus [3]. There is evidence for a changing distribution of some Phlebotomus spp. in Europe including P. ariasi, P. mascittii, P. neglectus, P. perfiliewi, and P. perniciosus, which are expanding towards more northerly and higher altitude places, and this is associated with climate change [4]. Phleboviruses are classified into three serological complexes: (Sandfly fever) Naples - phlebovirus (SFNv), Sicilian phlebovirus (SFSv) and Salehabad virus (SALv). SFNv has been isolated from P. perfiliewi, P. perniciosus, P. longicuspis, P. papatasi, P. sergenti and Sergentomyia minuta. SFSv and Corfu virus from P. ariasi, P. papatasi, P. neglectus, P. perniciosus and P. longicuspis. Salehabad species have been detected and isolated from P. perniciosus and P. perfiliewi [5].
Ecological plasticity
Sand flies naturally feed on blood from a wide range of mammalian and avian host species and are traditionally found in large numbers in the rural environment close to animals and humans. However, they also have a remarkable ability to colonise modern landscapes [6] [7]. In southern Europe, the vectors of L. infantum have adapted to peri-urban residential areas containing detached houses with gardens and dogs (the domestic reservoir of the parasite). It has also been found in high density close to blocks of flats in the town of Fuenlabrada in southern Madrid, causing the largest human outbreak of leishmaniasis in recent times on this continent [8]. As ectothermic insects, the large-scale territorial boundaries of sand flies are constrained by climate, particularly minimum winter temperatures affecting larval survival and cold and rainy summers that reduce adult biting activity [2] [9]. Tolerance to extreme temperature and precipitation varies between species and genetic lineages of the same species. For example, P. perniciosus is adapted to a broader range of climatic conditions than P. ariasi, which thrives in cooler and more humid environments [10] [11]. Phlebotomus sergenti strains from Morocco are less tolerant to low temperatures than those from Spain, which are considered better suited to potential colonisation of cooler areas in Europe [12].
Biting and disease risk
Data on the biting rates of sand flies affecting humans and animals are scarce. Since adult females are the only hematophagous stage, biting rates in a particular area would be strongly correlated to the abundance of adult females. Sand flies require a minimum of six weeks to complete a life cycle, with adult activity and, consequently, parasite transmission being mostly nocturnal and typically seasonal [2]. The active season for adults in Europe spans from April to November depending on the latitude, with warmer regions having longer seasons and up to three generations between May and September [2]. Areas with longer activity periods have a greater incidence of sand fly-borne pathogens. Sand-fly-endemic areas where a suitable vertebrate reservoir host species of the parasite is absent or rare may have high vector density and biting rates but may not sustain Leishmania transmission. An example of this is a peri-urban zoological park in southern Spain where most sand flies feed on large herbivores, which are not Leishmania reservoirs [13]. On a broader geographical scale, L. major is absent in Europe despite the vector P. papatasi being widely present, because there are no suitable rodent reservoirs for the parasite [14].
Geographical distribution
Brief history of spread and European distribution
The geographical distributions of sand fly vector species in Europe and neighbouring countries differ markedly among species and have been recently updated (https://www.ecdc.europa.eu/en/disease-vectors/facts/phlebotomine-sand-flies). Table 1 describes the distribution of sand fly vectors by country and region. Broadly speaking, P. perniciosus and P. ariasi are most abundant in western Europe including Portugal, Spain and France, and they are also present in northern Africa. P. perniciosus is the predominant vector, except in colder and wetter areas of south east France and the Pyrenees where P. ariasi is the dominant species. Phlebotomus perniciosus is also the main L. infantum vector in Italy, Malta and in many areas of the Balkan countries. Its eastern and northern limits are the Crimean Peninsula, western Germany and southern Switzerland, respectively (Table 1). South-east France and Italy represent the western limits for P. perfiliewi and P. neglectus, respectively. Their distribution reaches Hungary in the north (P. neglectus is also found in areas of southern Germany and Switzerland) and they are widespread in eastern Mediterranean countries. Phlebotomus perfilewi is also found in most North African countries. Phlebotomus tobbi and, to a lesser extent, P. similis and P. simici, are also present on the Balkan Peninsula, in Greece, the Middle East and Turkey. Phlebotomus mascitii is the species displaying the greatest longitudinal dispersion in Europe. It is generally found at a low density as far north as northern France, Belgium and Germany, to the west in north-eastern Spain and is endemic in some Balkan countries, Greece, Turkey and the Middle East. Phlebotomus alexandrii is reported in low density across the Iberian Peninsula, North Africa, Greece and Turkey. Other vectors in Europe and neighbouring countries are P. kandelakii, P. halepensis and P. balcanicus in Georgia, North Africa and the Middle East, P. langeroni and P. longicuspis in Spain and North Africa. Phlebotomus papatasi and P. sergenti are present in all European Mediterranean countries, North Africa, the Middle East and Asia (Table 1).
While minimum winter and summer temperatures are responsible for latitudinal differences in species distribution, wide temperature seasonality changes may partially explain the differences between eastern and western Mediterranean countries in terms of the spectrum of the species [9].
Future expansion
Vector distribution is sensitive to long-term changes in temperature and precipitation, leading to the occupation of new niches and abandonment of previous ones [15]. Statistical models have been developed to predict future climatically suitable areas for Phlebotomus spp. in Europe under various scenarios of global warming. Projections are based on climatic features in current vector distribution areas [9][16] and in one case they were complemented by probable migration routes as a result of taking into account landscape structure [16] [17].
Fischer et al. [17] predicted that P. ariasi will spread eastwards from its current western locations in France into western Germany and western Switzerland by 2040, and will continue along the border between these countries to reach south-eastern Germany during the period 2071-2100, and eastern and north-eastern Austria by the end of the century. An additional dispersion route for P. ariasi is predicted from Croatia and Slovenia into south-eastern Switzerland during the period 2071-2100. In 2041-2070, P. mascittii will disperse from its present locations in south-west Germany into north-eastern Germany and north-eastern Switzerland, from France into northern Switzerland and from southern Switzerland north to the river Danube and west along the Slovenian-Italian border. During the twenty-first century a gradual eastern spread is expected for P. perniciosus from south-west towards south-eastern Germany and from France into Belgium and western Germany. Phlebotomus neglectus and P. perfiliewi will disperse north from Italy and Slovenia (P. neglectus) to southern Switzerland and southern Austria by mid-century and possibly to eastern Austria by the end of the century. Phlebotomus neglectus was recently detected in southern Germany, but neither this species nor P. perfiliewi are expected to spread further north during the twenty-first century [17]. Predictions were similar for two climate change scenarios: the A1B Representative Concentration Pathways (RCP) scenario and the B1 scenario of the Special Report on Emission Scenarios (SRES), detailed in the fourth Assessment Report (AR4) by the Intergovernmental Panel on Climate Change (IPCC).
Using the A1B RCP scenario, Trájer et al. [18] predicted that the latitude boundary of suitable climatic conditions for P. ariasi will move from the present 49°N to 53°N latitude, reaching southern UK and 15°E, to the German-Polish border, Hungary and Turkey in the 2011-2040 period. Poland, Czechia, Slovakia, Romania, Moldova and Ukraine will become climatically suitable in 2041-2070. The areas for P. perniciosus will be similar to those for P. ariasi, with a lower likelihood of dispersion into Germany, Poland, and Czechia. Suitable areas for P. sergenti, P. similis, P. neglectus, P. papatasi, P. perfiliewi and P. tobbi, are not expected to greatly expand northwards. Suitable areas for Phlebotomus sergenti and P. similis will include Portugal, Albania, Romania, and Ukraine by 2070. Suitable areas for the other four species will encompass the Carpathian Basin, the Romanian Plain, the Balkans, Turkey and the Iberian and Apennine Peninsula. In addition, models predicted an increase in the period of vector activity in countries such as Spain and Greece, but not in Hungary [18].
According to the IPCC’s fifth Assessment Report, using the most recent predictions on climate habitat suitability based on the worst case scenario of greenhouse gas emissions (RCP 8.5., IPCC) [9], in 2061-2080, suitable areas for P. ariasi and P. mascittii would be restricted to northern Europe (UK, Scandinavia and the North Sea coast). Under less extreme RCP scenarios, habitat in Central Europe will still be adequate for P. mascittii. Models also predict increasing habitat suitability for P. perniciosus towards the north over time. Overall, expansion is projected for P. alexandri, P. neglectus, P. papatasi, P. perfiliewi and P. tobbi, decline for P. ariasi and P. mascittii and shift for P. perniciosus and P. sergenti.
None of these models considered other environmental factors that could affect vector dispersion, including extreme weather conditions such as flooding, and modifications in land use and cover, which are also subject to political and economic decisions. Failing to consider these influential factors leads to added uncertainty in the projections, particularly on a small geographical scale [17].
Entomology
Species name/Classification: Phlebotomus spp. (Diptera: Psychodidae)
Common name: Sand fly
Morphological characters and similar species
Of the five psychodid subfamilies, only the sand flies (Phlebotominae) have piercing mouthparts capable of taking blood. The phlebotomines tend to have an elongated and more fragile structure, in contrast to the squatter and more robust appearance of the other psychodid flies. Sand flies are tiny insects, 1.5-3.5 mm in length, with a hairy appearance, large black eyes and long, stilt-like legs. They can be distinguished from other small flies by their wings, which are hairy and extend at a 40° angle over the body when the fly is at rest or blood-feeding. Morphological identification of phlebotomine sand flies to species level is difficult, usually requiring examination of internal structures [19] [20].
Development and life cycle
Sand flies have a four-stage life cycle: egg, larva, pupa and adult. Eggs hatch after 4-20 days, although this is likely to be delayed in cooler weather [20]. Larval development involves four instars, and is completed after 20-30 days depending on species, temperature and nutrient availability. Environmental extremes (e.g. heat, cold, or drought) can cause larvae to diapause, prolonging development time for months. Larvae are mainly scavengers, feeding on organic matter (e.g. fungi, decaying leaves, animal faeces and decomposing arthropods). The pupal stage lasts 6-13 days before the adult sand flies emerge.
Adult feeding behaviour
Both male and female sand flies feed on plant juices and sugary secretions. Females also blood-feed to produce eggs. Sand flies use their mouthparts to probe exposed skin, leading to the formation of a pool of blood from which they feed. Sand fly saliva contains pharmacologically active components that aid in the feeding process [21]. Feeding activity is influenced by temperature, humidity and air movement (sand flies are weak fliers so even light wind can inhibit flight and reduce biting). Most species feed at dusk and during the night, when temperature falls and humidity rises [22], although daytime biting can occur indoors in darkened rooms or among shaded vegetation/trees, especially if disturbed by human activity.
Reproduction and oviposition
Adult sand flies mate soon after emergence; males locate females at resting sites or on vertebrate hosts, with the aid of pheromones [23]. Female sand flies usually lay 30-70 eggs during a single gonotrophic cycle, which are deposited in cracks and holes in the ground or in buildings, animal burrows and among tree roots [20]. The eggs require a microhabitat with high humidity in order to survive, but are not laid in water. Generally, one blood meal results in the production of a single batch of eggs [22].
Activity (incl. dispersal, seasonality, overwintering)
The extent to which sand fly population densities vary throughout the year depends on the local climate, with significant seasonal changes in temperature and precipitation resulting in fluctuations in sand fly numbers – i.e. lowest during the coldest and/or driest seasons of the year [22]. In tropical areas, some species are common all year, whereas others show marked changes in relation to wet and dry seasons. In temperate regions adult sand flies are only present during the summer. In addition, the various species of sand fly have different seasonal activity periods and daily peaks of biting activity. Adult sand flies are weak fliers, travelling with a characteristic short hopping flight, and they usually disperse no more than a few hundred metres from their breeding sites. Most species fly horizontally near ground level. In temperate and arid regions, sand flies may overwinter as diapausing mature larvae [20].
Habitat preference
Phlebotomus spp. occur predominantly in warm, humid, tropical climates and semi-desert vegetation habitats, although a few species occur in temperate zones. They are able to colonise rural, peri-urban and urban areas. Sand flies require a humid microclimate in order for their eggs to develop and larvae need a cool, moist habitat with decaying debris. Adult sand flies often inhabit rock crevices, caves, and rodent burrows, and in peri-domestic settings rest in cool, dark and humid corners of animal shelters or human dwellings [22] [24]. Both rodent burrows and peri-domestic areas provide ready access to blood meals in addition to shelter from the elements.
Host preference
Female sand flies feed on a wide variety of vertebrate hosts, including humans, livestock, dogs, urban and wild rodents, reptiles, amphibians, and birds [13] [20] [24]. Many species of sand fly are opportunistic and feed on those animals to which they have easiest access, as the same species collected from different biotopes often display different feeding patterns [25]. A study of sand fly species from farms and kennels in Italy found that P. papatasi, P. perniciosus and P. perfiliewi fed primarily on the host species (livestock and humans) that were present at the collection site [26]. If many different animals were present, both P. perfiliewi and P. perniciosus were found to feed on all of them [26]. Similarly, at a wildlife park in Spain Phlebotomus perniciosus, P. ariasi and P. papatasi fed on a wide range of species, however, there was evidence of preferential feeding on certain host species [27]. Feeding probability was also positively associated with increasing host census and decreasing movement costs of sand flies to the host [27]. As a result, it is probable that in urban and peri-urban settings humans and domestic dogs are the main targets for sand flies.
Epidemiology and transmission of pathogens
Vector status of different diseases in terms of public health and veterinary importance
Vector competence* refers to the ability of a vector to transmit a disease [28]. Experimental transmission studies in animals have implicated P. perniciosus and P. ariasi as competent vectors of L. infantum. A species may otherwise be considered a vector if it is attracted to a particular Leishmania reservoir host species and the same parasite strain is detected in the vector and the host in disease foci [29] [30]. Based on these criteria, species with confirmed vector competence for L. infantum include P. ariasi [31], P. balkanicus [32], P. halepensis [32], P. kandelakii [32], P. langeroni [33], P. neglectus [34] [35], P. perfilewi [36], P. perniciosus [37] [38] and P. tobbi [39] [40]. Suspected L. infantum vectors include P. alexandri [41], P. mascitti [42] [43] and P. major variants other than P. neglectus [44] [45]. Confirmed vectors of L. major and L. tropica are P. papatasi [46] and P. sergenti, respectively [47] [48]. Phlebotomus similis, a sister species of P. sergenti, has been implicated as the vector for L. tropica in Crete [49] [50]. Vectors of L. major and L. tropica are considered ‘specific vectors’ as they display strong specificity for these Leishmania species. In contrast, other Phlebotomus spp. support development of multiple Leishmania spp. and are termed ‘permissive vectors’ [51] [52]. Competence of Leishmania spp. vectors varies between species and is not fixed for a particular species as it depends on parasite-vector-host interactions as well as on the ecological and epidemiological features of the infection [30].
Traditionally P. papatasi was the implicated vector of phleboviruses. However, in the last decade, viruses have been detected in P. perfiliewi, P. perniciosus, P. longicuspis, P. papatasi, P. sergenti and Sergentomyia minuta, indicating sand flies have low specificity for these viruses. Infection is found in males and females suggesting transovarial and venereal transmission during mating [53] [5].
Factors driving/having an impact on transmission cycles
Risk of sand fly-borne pathogens establishing transmission cycles in non-endemic European regions
Vector-borne pathogens may not become established in a particular biotope if the vector population size and the activity period are too small (50 vectors/m2 sticky trap is considered the minimum density necessary for Leishmania transmission). This may explain the absence or low prevalence of L. infantum infection in areas of central and eastern Europe in spite of the presence of P. perniciosus, P. perfilewi and/or P. neglectus vectors [54]. Conditions promoting vector spread, such as a warmer climate due to climate change combined with the introduction of infected dogs, are considered the reasons for a northward expansion of P. perniciosus, P. ariasi and P. neglectus and canine leishmaniasis in northern Italy and Spain and southern France [55] [56] [57] [58] and for P. perniciosus being reported at higher altitudes in southern Spain [59].
The introduction of infected dogs in Leishmania-free areas poses the risk that leishmaniasis may become established in sand fly marginal areas, and there is an influx of infected dogs into northern Europe as a result of dog adoption schemes and tourism [60] [61] [62]. Furthermore, canine leishmaniasis due to congenital parasite transmission has been reported in three generations of dogs living in the Czech Republic [63]. Congenital transmission is considered an important infection route in the USA [64].
The absence of CL in southern Europe caused by L. major, despite the widespread abundance of its vector P. papatasi, is due to the lack of a suitable rodent reservoir host for these species [14]. Nevertheless, an L. infantum/L. major hybrid was detected in AIDS patients in Portugal which was shown to develop and enable transmission in P. papatasi [65] [66]. Leishmania major DNA was later detected in the sand fly Sergentomyia minuta [67], the natural vector of Leishmania (Sauroleishmania) tarentolae in reptiles. These findings highlight the risk of L. major introduction from North Africa and the potential for hybrids forming and spreading across the natural P. papatasi range, emphasising the need for continuing surveillance [66].
The establishment of the L. tropica cycle in southern European countries where the vector P. sergenti is widespread is a possibility since numerous cases are being diagnosed among people migrating into Europe from endemic countries [68]. Leishmania tropica was highly endemic on the island of Crete until the 1950s and cases have been continuously reported there and on the Ionian Islands since the early 2000s [69]. The suspected vector in Crete is P. similis, a species found in Greece and Asia Minor [49] [50]. Leishmania tropica also has a zoonotic cycle that relies on rodent species from North Africa and the Middle East which are not present in Europe.
Cyprus is the only area in Europe where cases of anthroponotic VL caused by L. donovani have been reported. This species has the largest public health impact worldwide, being endemic in eastern Africa and on the Indian continent and present in Turkey [70]. Leishmania donovani is closely related to L. infantum and an L. infantum/L. donovani hybrid successfully developed in P. tobbi and P. perniciosus [71]. Preventing further spread of L. donovani into Europe is considered a priority [69].
No studies are available on the risk of phlebovirus transmission cycles becoming established in non-endemic European regions.
Factors driving sand fly-borne pathogen transmission in endemic areas
The transmission intensity level in endemic areas is strongly seasonal and varies on a small and a large geographical scale, depending on environmental factors that affect vector and reservoir host abundance. The incidence of clinical cases depends on the pathogen incubation period which is at least two or three months for VL but only a few days for phleboviruses. The incidence of sand fly fever with the Naples and Sicilian viruses begins in April and reaches a maximum by September, closely mirroring the lifecycle of P. papatasi [46]. Neurological infections by Toscana virus occur during the summer months. In contrast, VL clinical cases in dogs and people in Europe often peak in the autumn and winter. Sand fly infection rates with L. infantum in the Fuenlabrada outbreak were highest at the end of the transmission period, indicating a higher risk of infection for people in late summer [72].
The risk of Leishmania infection depends on the density of infected vertebrate hosts and, apart from dogs, many domestic and sylvatic host species carry long-lasting subclinical infections which can potentially be transmitted to the vector [73] [74]. Several host species other than humans can become infected with phleboviruses but viremia is short-lived and no vertebrate reservoir host has yet been recognised [53]. This is epidemiologically puzzling because although viruses are transmitted congenitally in the vector, infection rates in subsequent generations gradually decrease, indicating that the vector alone is unable to maintain the viruses in the population permanently [46].
An illustrative example of the small-scale heterogeneity in the spatial distribution of sand fly-borne pathogens was the study of canine leishmaniasis in a 103 km2 highly endemic and geographically diverse rural municipality in Spain on the south-east Mediterranean coast [75]. Leishmania seroprevalence ranged from 0% in low (<100 m) irrigated colluvial farmland to 47-100% in the highest (157-362 m) and driest part of the municipality where the soil was predominantly conglomerate crust, favourable for the breeding and resting of sand flies. Dogs sharing a home with other infected dogs were at much greater risk of being infected than other dogs [75]. Elsewhere, a positive association between altitude and the incidence of leishmaniasis and vector density has been shown to exist [76] [77] [78] [79] [80]. Suitable altitude ranges differ, as they are an indirect measure of temperature, humidity, predominant wind, terrain characteristics and land use, all of which are critical factors affecting sand fly abundance and pathogen transmission.
Human intervention can strongly affect vector and parasite abundance. The recent L. infantum VL and CL outbreak in Fuenlabrada was triggered by disturbance of the natural habitat of P. perniciosus [81]. Vector density rose to >1000 vectors/m2 sticky trap in some areas following a demographic explosion of hares (Lepus granatensis) on which the vectors fed, with the hares acting as an atypical reservoir for L. infantum. Hares had found ideal breeding grounds in green leisure parks built around a newly urbanised agricultural area, occupied by a mostly urban human population, supposedly with a low level of acquired immunity against the parasite. Comparable scenarios occur when non-immune people enter CL and VL endemic areas, as was the case in situations with immigrant agricultural workers in North Africa and the Arabian Peninsula, communities displaced by war in Sudan, and American and European soldiers deployed in conflicts in Syria, Iraq and Afghanistan [82]. Similarly, absence of acquired immunity against phlebovirus infections led to thousands of clinical cases during World War II among soldiers stationed in the Mediterranean and the Middle East [46].
Changes in vector density leading to an increase in transmission potential were also associated with recent epidemics of VL, affecting hundreds of local adults and infants in endemic regions in Italy [83]. The causes of these epidemics remain unclear and, as in the Fuenlabrada outbreak, they were not associated with greater disease incidence in dogs. They were characterised by a generalised expansion of transmission foci rather than a few large case clusters, with P. perniciosus being the most common vector, followed by P. perfiliewi and P. neglectus [83].
Immunosuppression among HIV-positive patients was the determinant for the Leishmania-HIV virus coinfection epidemic of the late 1980s and 1990s in Mediterranean countries. There was no evidence of increased exposure to infected sand fly vectors, rather the sharing of needles among HIV+ intravenous drug users was established as a new L. infantum transmission route [84]. The Leishmania-HIV virus coinfection epidemic was the first major epidemic of human leishmaniasis in Spain since the 1960s. Prior to this, leishmaniasis was a common disease in humans in Spain, particularly among infants lacking acquired immunity, and it was associated with malnutrition, poor sanitation and the Spanish Civil War [85]. In western European countries, leishmaniasis is no longer a disease which mainly affects children. In contrast, this age group is still the most vulnerable in Albania [35]. Poverty and conflict are major determinants for the emergence of CL and VL cases in war-ridden Iraq, Syria Afghanistan and Sudan since the 1980s and 1990s, associated with population displacement into endemic areas, destruction of healthcare infrastructure and increased incidence of other diseases [82] [86].
Public health control measures
The suitability of leishmaniasis control strategies depends on the epidemiology of infection and the ecological characteristics of the vector and reservoir hosts, differing substantially for zoonotic and anthroponotic leishmaniasis. At present, there are no nationwide surveillance and control schemes for sand flies in southern Europe. Leishmania infantum control in Europe largely relies on preventing vector infestation in dogs using pyrethroid insecticide-impregnated collars and spot-on applications [87] [88]. The efficacy of these products under laboratory conditions is close to 90% and experimental field studies in dogs have demonstrated their usefulness in reducing leishmaniasis in people and dogs [89] [90] [91] [92] [93] [94] [95]. They require strict adherence to the recommended application frequency, they must be used throughout the entire vector season and their efficacy at a population level depends on the proportion of treated dogs and the infection incidence, with collars being more useful when incidence is high [89] [96]. Common problems causing reduced efficacy include collar losses, inappropriate mechanical application of topical solutions and product wash off when the skin is soaked in water. For these reasons, the effectiveness of insecticide preventive treatments in dogs may be very limited when left to dog owners in uncontrolled, non-experimental conditions [97].
Public health interventions to control leishmaniasis outbreaks or foci require an integrated strategy, combining vector surveillance and action against vector and reservoir species [86] [98] [99]. The Fuenlabrada human leishmaniosis outbreak of L. infantum transmitted by P. perniciosus, represents the only recent example in Europe where this approach was used [100] [101] [102] [103]. There were four main aspects to the programme: vector surveillance; environmental interventions to remove potential vector breeding and adult resting sites; large-scale environmental application of insecticides, and drastic reduction of the population of hares and rabbits, reservoirs of Leishmania.
Surveillance aims to assess the risk of parasite transmission and guiding vector control activities. Due to the difficulty in locating sand fly breeding sites, it centres on monitoring the distribution and abundance of the adult stages [98] [99]. A variety of sampling methods are available [24], the most common being sticky interception traps to capture a random selection of the sand flies flying in the immediate surroundings, light traps (with/without CO2 baiting) to catch host-seeking females attracted to light, and aspirator collection to catch resting flies. Emergence traps can be used to catch flies as they leave resting sites such as animal burrows. Animal-baited traps are another option useful for sampling host-seeking flies [24]. Weather conditions such as temperature, humidity and wind speed can affect sampling success. Selected sampling sites in Fuenlabrada were peri-urban parks and the urban infrastructure close to the residences of leishmaniasis cases where transmission was considered to be occurring.
Environmental interventions to eliminate sand fly breeding and resting sites in Fuenlabrada included removing organic waste, unwanted vegetation, wood piles and waste tips; cleaning drainpipes and watercourses; sealing sewage drains and electricity meters; plastering holes and cracks in the walls of buildings; placing wire mesh in the windows of sanitary basements and aeration tubes; eliminating rats and feral cat colonies and destroying hare and rabbit nesting grounds. Pyrethroid insecticides were applied on vegetation and water collection drainage systems in parks and urban areas close to leishmaniasis cases, around subterranean electricity networks, on walls covered with vegetation and in the sanitary basements of buildings.
Control activities in Fuenlabrada reduced the incidence of human leishmaniasis from 34.03 per 100 000 people in 2011 to 3.41 cases per 100 000 people in 2017 (incidence was 0.81/100 000 people in 2001) [104]. Culling of hares was the single most effective measure reducing incidence of leishmaniasis by 56% over two consecutive seasons. Elimination of potential sand fly breeding and resting sites was also considered effective in reducing sand fly density. In contrast, widespread application of insecticides on the environment was short-lived and unexpectedly, sand fly trap counts rose after the insecticides were applied, presumably due to an increase in vector activity and vectors evicting treated resting sites.
Eliminating reservoir hosts to control zoonotic leishmaniasis has been attempted before. Leishmania major was successfully eliminated in areas of the former USSR in Central Asia by destroying the natural habitat of rodent reservoirs and this approach is now being applied in the Middle East and North Africa [82]. In contrast, the culling of infected dogs in Brazil between 2008 and 2017 did not reduce zoonotic human VL from L. chagasi (syn. L. infantum) due to low coverage and low sensitivity of diagnostic tests. The measures also met with widespread criticism for ethical reasons [105] [106].
Insecticide spraying on sand fly resting sites has shown contrasting results in other countries. Sand fly density in tropical rainforests was reduced by 30% in Panama using the organophosphate malathion [107], whilst in Brazil no sand flies were observed for 21 days after the application of the organochlorine DDT [108]. Spraying cyfluthrin inside termite mounds and animal burrows in Kenya decreased sand flies by 90% for two weeks [109]. Continuous insecticide spraying against the malaria mosquito vector with DDT in the mid-twentieth century significantly reduced the incidence of Leishmania and phlebovirus infections in Europe and India [69] [110] [111]. Fipronil insecticide oral bait is being considered as an alternative to mass fumigation of rodent burrows for L. major control in Kazakhstan [112].
Attractive toxic sugar bait (ATSB) sprayed on vegetation or on sand fly barrier fences [113] has been used to reduce adult sand fly populations in Israel [114], Morocco [115] and Iran [116] where L. major is endemic. Common bait includes natural products such as boric acid, Spinosad and sodium ascorbate [114] [117]. ATSB is considered useful in arid areas where attractive flowering plants are scarce or absent, since it is more selective, environmentally friendly and less expensive than the large-scale use of insecticides [114]. In Israel, Bacillus sphaericus was applied to sugar solutions close to sand fly habitats as a means of biological control, causing significant larval mortality and reduction of the adult population [118]. Bacillus sphaericus reduced the survival and fecundity of sand flies in vitro [119] [120].
Ensuring the collaboration of the general public and medical and veterinary professionals is a key factor for the correct management of leishmaniasis control programmes and to reduce the risk of further outbreaks. Public health awareness and education initiatives and materials provide information on the disease and the vector and on personal protection against sand fly bites. The latter includes using insecticide repellents, fitting mosquito screens on windows at home, sleeping or resting in screened or air-conditioned rooms and protecting pets with insect repellents.
The participation of well-informed communities, coupled with active case finding by health authorities is critical to the success of integrated control strategies in areas where L. major, L. tropica and L. donovani are endemic [86]. Vectors of these species are also endophilic, and peri- and intra-domiciliary control measures are essential, particularly indoor residual spraying (IRS) with insecticides and the use of insecticide-treated bed nets (ITN) and linen [111] [86]. IRS is recommended when transmission incidence is high, but is operationally challenging and its efficacy must be assessed by observing the performance of people conducting the spraying; evaluating the accuracy of IRS; performing bioassays; monitoring knock-down catches of sand flies resting indoors, and assessing people’s acceptance of the intervention [86]. ITNs represent a very effective, low-hazard method for reducing human-sand fly contact and transmission of leishmaniasis in the population, as shown in trials carried out in many CL and VL endemic countries [121] [122] [123] [124]. However, achieving widespread coverage and enduring compliance in their use can be a major limiting factor [113] [125] [126].
Key areas of uncertainty
Environmental interventions to control vector populations need to be more clearly defined, based on a better understanding of factors leading to the large and small-scale spatial distribution of sand fly vectors, including breeding and resting sites. Further research is needed to improve the efficacy and understanding of the implications of insecticide treatment and to evaluate environmental persistence and application techniques, potential associated health risks and the development of resistance which may compromise the long-term effectiveness and sustainability of insecticide-based interventions [103] [127]. Strategies targeting sugar-feeding adult sand flies require wider knowledge of the vegetation flies feed on and to identify better attractants and ways of delivering them. The use of insecticide-impregnated vertical barriers in foci where sand flies reach human dwellings from the periphery could be further explored and standardised. Personal protection against sand fly bites could be improved by developing and testing novel sand fly repellents and ITN strategies [113]. With respect to the pathogens themselves, TOSV is not listed in international surveillance programmes, and this limits the timely availability of data on the incidence of this disease in the EU. Furthermore, there is uncertainty on the clinical impact and epidemiological characteristics of newly described phleboviruses and their interaction with Leishmania parasites in sand flies [46] [53]. Public health protocols to control the epidemic foci of sand fly-borne pathogens in Europe could benefit from standardisation and the results from interventions should be more widely communicated to the scientific community.
Footnote
*Vector competence is the physiological ability of a mosquito to become infected with and transmit a pathogen, and is typically assessed in laboratory studies. In nature, transmission of a pathogen by vectors is dependent not only on vector competence but also on factors describing the intensity of interaction between the vector, the pathogen and the host in the local environment. Therefore, vector and host densities, geographic distribution, longevity, dispersal and feeding preferences have to be considered to determine the vectorial capacity of a vector population and its role in transmission
Further reading
There are no open-source textbooks to introduce sand flies to a wider audience. The following documents are recommended:
Maroli M, Feliciangeli MD, Bichaud L, Charrel RN, Gradoni L. Phlebotomine sand flies and the spreading of leishmaniases and other diseases of public health concern. Medical and Veterinary Entomology. 2013 Jun;27(2):123-47.
Ready PD. Biology of phlebotomine sand flies as vectors of disease agents. Annual Review of Entomology, Vol 58. 2013 2013;58:227-50. doi: 10.1146/annurev-ento-120811-153557
Dvorak V, Shaw J, Volf P. Parasite biology: the vector. In: F Bruchi, L Gradoni, eds. The Leishmaniases: old neglected tropical diseases. Cham, Switzerland: Springer; 2018. pp. 31-76. ISBN: 978-3-319-72385-3.